World Quantum Day: Adventures in Quantum Control
A small journey together, to cook eggs, program quantum controllers, and definitely not send any carrier pigeons.
In our messy physicist heads, every day is about quantum. But today is different. Today, experts and enthusiasts from over 65 countries align with a common goal: promoting Quantum Science and Technology around the world. So this one’s for you, Mr. General Public. I intend to give you a peek into Quantum Control and, with a bit of luck, some insight into why we care so much about it. I also invite you to check out World Quantum Day and their awesome initiative!
We’ve had a fun ride from the first quantum revolution to this day, on the cusp of the second. Today we’ll see why controlling individual quantum systems, e.g. atoms or ions, is so crucial for our future. But first, let me give you some context about our past and present.
How It Started: History Check
Are you familiar with atomic clocks, lasers, and transistors? You know, the inventions that gave us GPS, fast internet, and computers? These technologies exist thanks to the first quantum revolution in the 20th century. These innovations were groundbreaking. I guess that’s why they call it a revolution: it turned the world upside down within a decade or so.
One thing that all those technologies have in common is that they focus on the behavior of a large ensemble (macroscopic) of quantum systems. Instead of addressing a specific atom, ion, or photon individually, we care about the average atom, ion, or photon. Those ensembles interact only moderately with the environment, and, at a macroscopic scale, they are easy to handle. Any single atom can do weird things. Since the useful properties rely on the average behavior of a very big pool, we don’t lose much in weirdness. Trusting a single atom to be stable and reliable, now that’s a whole other thing.
How It’s Going: Why We Should Care
Some say we are now living in the early stages of the second quantum revolution. Exciting, right? Contrary to the previous one, this new technological jump is about the state of individual (microscopic) quantum systems as part of an entangled and carefully engineered collective, not just any pool. A well-defined group of atoms can have states which are non-classical superpositions. That is where things start getting weird, and our intuition becomes fuzzy. You know, like the famous cat being both dead and alive. But we’re not here for a theoretical physics course, are we? So what do we do with those fancy entangled systems?
We don’t yet know the full extent of the influence a large quantum computer will have. Still, with a big enough machine, we expect significant changes in cybersecurity, optimization problems, and potentially groundbreaking results in many fields, from medicine and chemistry to AI and many more. This second revolution has all the right cards to become as groundbreaking as the first. Could this mean the cure for cancer, computers more intelligent than humans, and the colonization of Mars? I have no idea! Probably not right away, but this new technology will be disruptive and not just incremental.
Personally, I hope to see some solutions, within my lifetime, to the big challenges of our times, such as global warming and sustainable energy production. To the interested reader, I suggest the recent work of Fedorov et al. [1] to go deeper into the quantum advantage and its applications. It’s best to read about prospects from the people working in the field. Otherwise, it’s far too easy to find hyped-up and unsubstantiated claims. For now, all we need to know is that quantum computers promise to change our lives in major ways.
To get there, we need to take a dive into control systems for quantum computers. This will help us understand why the box performing the operations on a quantum system is a challenging yet critical ingredient of the second quantum revolution.
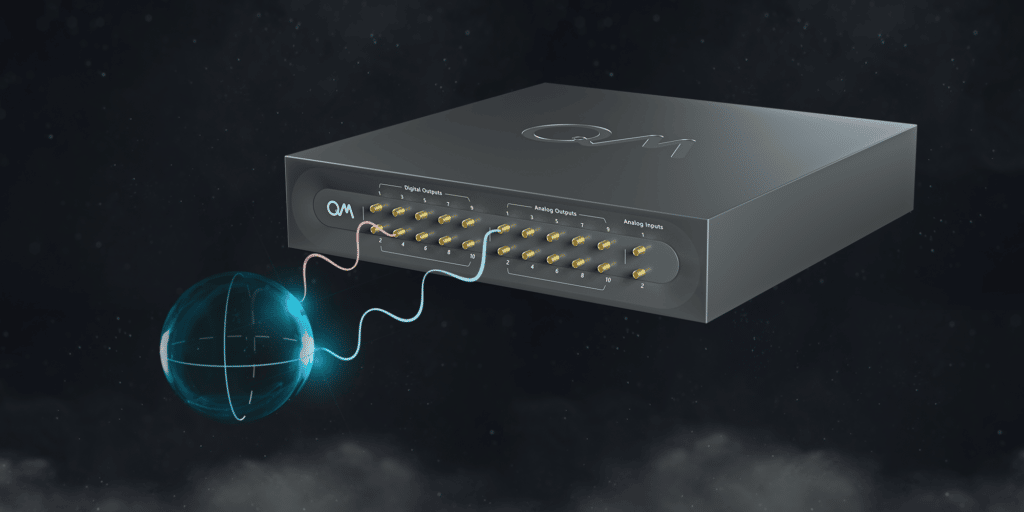
Quantum Control: What It Is and Why It Matters
Both classical and quantum computers use logic gates to process data. But quantum logic operations are fundamentally different. Classical gates consist of physical entities of wires and transistors embedded in the processor. Quantum gates, on the other hand, require a separate classical controller to generate electromagnetic pulses and send them into the quantum system.
The quantum gates are operations performed on the qubits (quantum bits) and change their state thanks to a series of such pulses (get an idea from Fig.1). Think of it as an egg in a microwave: some magic happens, and you only know it happened because the egg is now warm: it’s in a different state.
Additionally, the state of our qubits only lives for a very, very short time. That’s because individual quantum systems are so wildly sensitive. So while the bits in your hard drive are stable virtually forever, talking about stability for qubits is like saying you never smoke in between cigarettes. It might be technically accurate, but it’s of little importance.
Classical computers rely on our ability to fabricate the hardware components to make gates. Quantum computers, however, require a control system capable of decision making and performing gates in the extremely short timescales in which our qubits’ lives are stable. We must perform all operations and decisions from one cigarette to the next. Just to give you an idea of the timing, a common one-qubit operation called the NOT-gate takes approximately 20 nanoseconds for some qubits. If we look at a single second as the age of our planet, from formation until now, these 20 nanoseconds would be the time it takes you to blink. That’s how short 20ns is.
This is why making useful quantum control systems is darn hard. It’s also why quantum control is virtually as crucial as the quantum processing units themselves. There is no quantum computer without a quantum control system.
A Whole New “Think Fast and Slow” Paradigm
We now roughly understand how crazy fast a quantum control system has to be. The problem is that to really live the quantum dream, this box also needs to be smart and flexible.
In the early days of classical computers, people used static memory outputting to digital-to-analog converters. This was some very inflexible memory containing the instructions regarding a certain operation. Trying this route for quantum computers would be like counting grain bags by engraving inscriptions in stone, as our Egyptian ancestors did. Sure it can work for some specific application, but anyone can tell you this is not living the dream.
In the modern age of classical computers, we use generic CPUs. Those ultra-flexible smart components can make any calculation and take any decision. Unfortunately, those electronic marvels are not fast enough for quantum computing. It would be like trading in the stock market by placing orders using carrier pigeons. It’s a step up from stone engravings, and it works to an extent, but you can’t expect to make a lot of smart moves this way.
Today’s best bet for a practical quantum controller is field-programmable gate arrays (FPGAs). Those electronic components are challenging to program. Still, their clock cycles are of just a few nanoseconds; that’s even less than a blink compared to the Earth’s age units from the previous analogy. Such short clock cycles allow performing any smart classical computation in qubit-times. It also facilitates generic feedback operations. You can read the state of your qubit and decide what to do next based on the result. Imagine the difference between strictly following a recipe while making dinner and being able to taste and adjust it while cooking. Additionally, FPGA processors massively speed up the execution of parameterized dynamic circuits. This allows operations to change dynamically in a fast and flexible manner.
Quantum Future: The Challenging Road Ahead
Unlocking the full potential of full-fledged quantum computers is an immense challenge. A fast and flexible quantum control architecture is a requirement for any attempt to speed up the timeline towards practical applications of quantum systems. The ideal quantum control architecture must combine the flexibility of the fastest classical components with the power of the best qubits, and remove all operational barriers to ease of use.
If you want to read more about quantum control, its challenges, and possibilities, I strongly suggest diving deeper with Lior Ella in his recent article on IEEE Explore [2].
Such control systems have to be built with quantum applications in mind. Programming and job execution should be intuitive and straightforward. Calibrations and maintenance should be no-brainers. From the software you use to write your ideas to the processor that runs them, every piece of the chain must be dedicated to quantum experiments.
At the time of writing, quantum computers are not yet able to outperform the best classical computers on tasks of practical importance. A quantum advantage over classical computers has already been achieved for some specific tasks [3-4]. This seemed like a distant dream only a decade ago. We have yet to find a limit to the marvels we can achieve while working together. I am certainly excited to see what comes next! This is why I’m a big fan of initiatives like World Quantum Day as a means of sharing our ideas and passion, aiming at building a better future together.
References
[1] Fedorov, A. K., et al. “Quantum computing at the quantum advantage threshold: a down-to-business review.” arXiv preprint arXiv:2203.17181 (2022).
[2] L. Ella, “How to Build a Scalable Quantum Controller,” in Computer, vol. 55, no. 3, pp. 91-94, March 2022
[3] Arute, Frank, et al. “Quantum supremacy using a programmable superconducting processor.” Nature 574.7779 (2019): 505-510.
[4] Zhong, Han-Sen, et al. “Quantum computational advantage using photons.” Science 370.6523 (2020): 1460-1463.